The Brouard group
Gas phase reaction dynamics and imaging mass spectrometry
The Department of Chemistry, Oxford University,
Oxford, United Kingdom
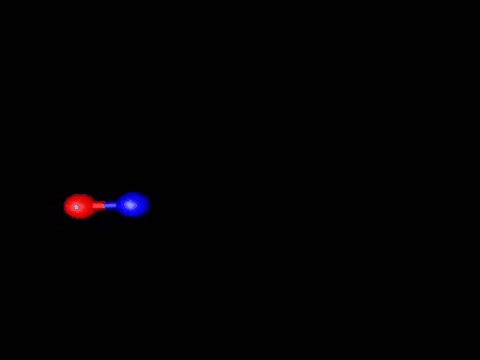
Our research
A Brief Overview
The research conducted by the group encompasses several different fields. Our lab currently contains 4 seperate experiments each involved in one of our key focus areas. These experiments fall under three main categories: ![]() |
|
Reaction Dynamics
Our research in reaction dynamics is currently focussed on state-selected inelastic scattering.
- Hexapole State Selected Scattering
Our crossed-molecular beam machine, also known as the Blue Monster, combines hexapole state selection, electric field orientation, and velocity-map ion imaging to obtain an explicit picture of the scattering dynamics occurring between NO molecules and a collision partner (currently rare gas atoms). The hexapole focuses supersonically expanded NO molecules in their ground rotational state, and the electric field, located in the centre of the scattering chamber, enables the spatial orientation of the molecules. This technique provides control over the part of the molecule the collision partner impacts on and we can distinguish, for instance, between end-on collisions taking place at the N- and the O-end by switching the direction of the electric field. The rotationally excited NO are resonantly ionized and velocity mapped onto a detector and imaged with a camera to obtain angularly resolved scattering distributions. These experimentally measured angular distributions can then be compared to those predicted by exact quantum mechanical scattering calculations.
![]() |
|
Our scattering studies allow for an extremely detailed
description of the collision dynamics and, in particular,
shed light on steric preferences associated with specific
quantum states and collision geometries. The measurements
provide valuable information about the underlying molecular
forces and the potential energy landscapes that govern the
dynamics, and are thus also a stringent test for the
validation of theoretical models.
The latest experiments on the Blue Monster have
investigated the three-vector correlation dynamics of end-on
and side-on oriented NO molecules with argon atoms. In
these experiments, we detect rotationally excited NO
molecules resulting from a collision with three well-defined
vectors; the initial NO bond-axis vector, r, and the initial
and final relative velocity vectors, k and k'. By recording
the direction of the outgoing relative velocity vector of
the system, we are able to distinguish between collisions
taking place on either side of the molecule. Our results
show striking differences in the differential scattering
distributions (or differential cross sections , DCSs)
between the two ends and between the two sides, and a
pronounced oscillation in steric preference as a function of
the final rotational state being probed. These oscillations
are a result of a quantum mechanical (QM) interference
effect.
![]() |
![]() |
Experimental and QM simulated images
for the N- and O-end orientations (first/second, and
third/fourth row, respectively) for the final
rotational states between 3.5e and 16.5e.
|
The Integral Steric
Asymmetry (ISA) for end-on collisions is plotted as a function
of the final rotational state, j'. A positive ISA
indicates a preference for N-end collisions, while a
negative ISA indicates a preference for O-end
collisions. The QM calculations reproduce the
experimental data very well, whereas the quasi-classical
(QCT) calculations fail to capture the alternation in
the sign of the ISA for adjacent j' transitions.
|
Photoinduced Dynamics
Our experiments in Photoinduced Dynamics are split into three different sub areas, described below.- Coulomb Explosion Imaging
When exposed to intense laser fields,
molecules may rapidly lose multiple electrons. The resulting
polycations are unstable and rapidly fragment into smaller,
mutually repulsive charged fragments - a so called "Coulomb
explosion". Tracking the momenta of these fragments allows
the original structure of the molecule to be determined.
This is done by multi-mass velocity map ion imaging using
the PImMS camera. The relative velocities of the
different fragments can be determined through covariance
analysis of the individual ion images, revealing great
detail about the original molecular structure and the
Coulomb explosion dynamics. This technique has been recently
used in collaboration with Henrik Stapelfeldt's group at the
University of Aarhus to identify structural isomers on a
femtosecond time scale.
-
Femtosecond Reaction Dynamics
|
Coulomb explosion imaging and covariance analysis. The covariance maps cov(A+,B+) show the velocity distribution of a given ion (B+) relative to a "reference" ion (A+). |
The group has recently purchased and
commisioned our own Ti:Sa femtosecond laser system. This
will be used in the near future for a range of studies
into Coulomb explosion dynamics and to explore potential
applications of Coulomb explosion imaging.
The use of femtosecond laser pulses allows
the study of photoinduced dynamics as they happen in
'real time'. Here, a 'pump' laser pulse is used to
induce the dynamics we want to study, and this is
followed by a intense 'probe' pulse, which ionizes the
system, and allows for the ionic fragments to be
velocity mapped. Varying the delay between these two
pulses allows the dynamics initiated by the 'pump' pulse
to be followed on a femtosecond time scale, creating a
'molecular movie'.
The structural information provided
through the measurement of ion trajectory correlations
has also been used as a probe to follow the induced
torsional motion between the two carbon rings in a
biphenyl molecule. The torsional motion is first
initiated using a femtosecond 'kick' laser pulse,
followed a short time later (ps) by a much more intense
'probe' laser pulse, which Coulomb explodes the
molecules. By measuring correlations between F+ and Br+
ion trajectories using the PImMS camera we were able to
follow the motion of the molecule out to picosecond
timescales, watching the two rings periodically rocking
back and forth.
![]() |
|
|
|
More recently, we have applied this technique to study
photodissociation reactions in real time in a range of
halomethane molecules. This work was done in collaboration
with Daniel Rolles and coworkers at the FLASH free-electron
laser in Hamburg. Here, a UV pump pulse initiated
dissociation of a C-I bond, before a Coulomb explosion was
induced by a probe laser, at a range of pump-probe delays.
At longer pump-probe delays, the two neutral fragments
originating from the photodissociation are further away from
each other upon ionization by the probe pulse, and thus feel
a smaller Coulomb repulsion. This channel therefore has a
kinetic energy release which decreases as pump-probe delay
increases. Analysis of this channel in the ion images allows
us to determine information about the timescale of the
dissociation, and how internal energy is partitioned
following it. Applying covariance analysis allows us to
determine the delay dependent relative ion momenta.
In the near future we hope to use this to study more complex
photochemistry, such as photoisomerisations, in real time.
![]() |
|
|
|
- 3D Imaging
By using the capibilities of the PImMS camera, combined
with home designed Newton-Sphere elongating ion optics we
can not only slice the Newton sphere to gain higher
resolution, but we can also achieve full 3D imaging of the
molecular fragments. By slicing the Newton sphere, and
centroiding in time and space, we are able to record full 3D
velocity distributions without resorting to mathematical
methods such as an Abel transform in order to obtain them.
By using this powerful techniqe, blurring from pairwise
correlations in diatom diatom inelastic scattering can be
all but eliminated and 3D images obtained even in cases
where the system lacks cylindrical symmetry.
![]() |
|
|
|
Imaging Mass Spectrometry
Imaging Mass Specrometry focuses on the efficient analysis
of biological samples and developing technologies in order
to perform this analysis. Our work in this area focusses on
microscope mode mass spectrometry, in which spatially
resolved chemical information about a surface can be
obtained at very high throughput.
Imaging mass spectrometry is a powerful technique we develop in order to answer several questions: Which molecules are present in a tissue sample? Are there specific molecular markers which identify tumour cells? Where in a biological sample are drugs located a certain time after ingestion?
We are specialists in the development of new ion optic
systems allowing imaging mass spectrometry to be performed
with enhanced instrument specifications. By defocusing an
ionisation laser onto a large section of a sample, molecular
ions are emitted from the sample and spatially mapped onto a
position sensitive detector. The observed ion image shows a
molecular map of the surface. By employing self developed,
ultrafast micro-channel-plate (MCP) scintillation detectors
in combination with CMOS based imaging sensors (via the
PImMS collaboration), we are able to detect multiple ion
images of various masses within a single experimental cycle.
![]() |
|
Work in the group has focussed on developing instrumentation to push the resolution limits of miscroscope mode mass spectrometry in the spatial and mass domains. Recently, a novel microscope-mode reflectron instrument has been developed, as shown schematically above. This, in addition to new pulsed extraction schemes has greatly improved the resolution at which we can image, and the m/z range the technique can be succesfully applied to.
![]() |
|
Collaborations
Meet some of our frequent collaboratorsFunding
We are grateful to the EU, STFC, and EPSRC for previous funding. We are currently funded through EPSRC Programme Grants in New directions in molecular scattering, and Ultrafast photochemical dynamics in complex environments.